Study suggests dynamic twists, loops can enable DNA to modulate its function
Generally when DNA is visualized, it's a string-like double helix structure which is thought of, however, the reality is that DNA double helix in cells is supercoiled and constrained into loops. Every aspect of DNA activity is known to be influenced by this supercoiling and looping, but how this happens is still unclear.
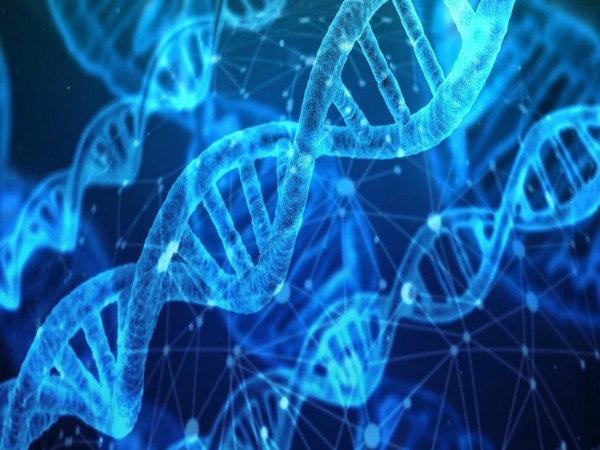
- Country:
- United States
Generally, when DNA is visualized, it's a string-like double helix structure which is thought of, however, the reality is that DNA double helix in cells is supercoiled and constrained into loops. Every aspect of DNA activity is known to be influenced by this supercoiling and looping, but how this happens is still unclear. Published in the journal Nature Communications, a study by researchers at Baylor College of Medicine shows that supercoiling and looping can transmit mechanical stress along the DNA backbone.
The stress can promote the separation of the strands of the double helix at specific distant sites, exposing the DNA bases, which may facilitate repair, replication, transcription or other aspects of DNA function. "DNA stores a cell's genetic information in a stable and protected form that is readily accessible for the cell to carry on its activities," said corresponding author Dr Lynn Zechiedrich, Kyle and Josephine Morrow Chair in molecular virology and microbiology at Baylor. "Organisms achieve this seemingly paradoxical goal by storing DNA in supercoiled loops. In the current study, we investigated how supercoiling and looping modulate DNA activity."
Zechiedrich and her collaborators began by making small pieces of supercoiled DNA, like those present in living cells. They took a short, linear DNA double helix and twisted it once, twice, three times or more, either in the direction of the turn of the double helix (positive supercoiling) or against it (negative supercoiling). Then they connected the ends together forming a loop. "In a previous study, we had looked at the 3-D structures of the supercoiled minicircles with electron cryotomography (cryo-ET), an imaging technique that produces high-resolution 3-D views of large molecules," said Zechiedrich, a member of Baylor's Dan L Duncan Comprehensive Cancer Center. "We observed a surprisingly wide variety of minicircle shapes depending on the specific supercoiling level. Many of the shapes we observed contained sharply bent DNA. This observation was unexpected."
It was unexpected because the models indicate that supercoiled DNA circles would behave more like a twisted rubber band. "We discovered that supercoiled, looped DNA, instead of gently bending, suddenly pops out sharp edges that produce a disruption in the double helix," Zechiedrich said. "The openings expose that particular DNA code, making it accessible to proteins looking for specific sequences to interact with the DNA, for instance, to repair it or make a copy of it."
"Another important finding is the idea of 'action at a distance,'" said first author Dr Jonathan M. Fogg, a senior staff scientist in the Zechiedrich lab. "The effects of supercoiling stress at one side of the loop can be transmitted along the DNA backbone to a distant site. For instance, if one site is sharply bent, a second site far away from the first site also will be sharply bent. Studying linear DNA does not capture this phenomenon, but our supercoiled minicircles reveal these dynamic properties of DNA as it is found in cells." These findings suggest a new perspective on how DNA activities are regulated. Currently, the idea is that specialized proteins interact with DNA to separate segments of the double helix that need to be duplicated, for instance, or transcribed into RNA to produce a protein.
"Here we showed that no protein is needed to access DNA, it can make itself accessible on its own," Zechiedrich said. "Our cells have created many complex processes to deal with storing and using DNA, and the shape of that DNA affects all of them," said co-author Allison Judge, a graduate student in the Department of Pharmacology and Chemical Biology.
"Our findings provide new insights into what governs DNA shape," said co-author Erik Stricker, a graduate student of paediatrics-oncology. "We propose that variations in these novel DNA shapes could have potential nanotechnology applications, such as gene therapy." "Our study rebrands DNA from a passive biomolecule to an active one," said co-author Hilda Chan, a graduate student in the Medical Scientist Training Program. "Our findings stimulate future work on how DNA may use its shape to govern access to specific sequences in a variety of situations, like in response to drugs, infection or points in the cell cycle." (ANI)
(This story has not been edited by Devdiscourse staff and is auto-generated from a syndicated feed.)
- READ MORE ON:
- Nature Communications
- Kyle
- Baylor College of Medicine